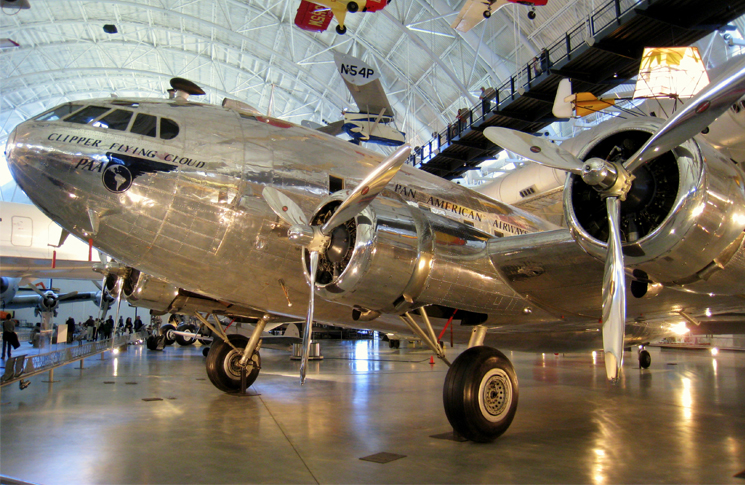
QNH/QFE
Quite why the letter Q is used in abbreviations for altimeter settings is a quintessentially curly question. The answer is in the radio Q codes that were chosen at the Third International Radiotelegraph Convention in London, in 1912. These were Morse code abbreviations for frequently asked questions. A section of the Q codes was allocated to aviation, thus QNH stands for barometric pressure adjusted to sea level. An aircraft altimeter set to QNH will show the elevation of the airfield. An aircraft altimeter set to QFE will show zero altitude. Aerodrome information services for pilots include QNH settings so pilots can correctly calibrate their altimeters for landing. Although Morse code has long been obsolete in aviation the Q codes remain, both as relics, and as unambiguous terminology. Some other points: QNE is the Q code for the standardised QNH setting of 1013.25 hectopascals that is used for setting altimeters to flight levels. And in the US QNH is expressed as imperial inches of mercury instead of hectopascals/millibars.
Radio
Radio and the aeroplane are about the same age and they came together before World War I when several independent pioneers experimented with Morse code transmission to and from aircraft. However a German Zeppelin, LZ-6, had already communicated with the ground by radio in 1909. By 1914 aircraft-to-aircraft radio communication had been demonstrated and the following year air to ground Morse radios were being used by British reconnaissance aircraft. By World War II voice radio was common in military use, with Morse code being reserved for long-range transmissions. In civil aviation, radio-enabled reporting of aircraft positions, allowed a primitive form of en-route air traffic control. Its major safety benefit in general aviation comes from alerted see-and-avoid. The combination of radio and looking out has been found to be eight times more effective than unalerted see-and-avoid. This stands to reason. You are far more likely to see an aircraft that has already told you it is around.
Radio altimeter
Like many inventions that have made great contributions to modern civil aviation the radio altimeter was invented early (in 1924) and first found widespread use as a weapon of war. The German Junkers 88 night fighter used one in World War II. A radio altimeter works on the same principle as radar: radio waves are directed at a target – in this case the ground. The time they take to bounce off the target and return allows the aircraft’s height to be calculated. The accuracy and freedom from barometric uncertainty of the radio altimeter makes it an essential part of automatic landing systems, and of terrain awareness warning systems (TAWS).
Runway standards
In the beginning any piece of flat ground was good enough for landing an aeroplane. But as aircraft and air transport have become more sophisticated, standards have evolved for runways. The ICAO-based Australian runway standards are found in Civil Aviation Safety Regulations Part 139 – the Part that covers aerodromes. These mandate aspects such as length, width, thickness of material and foundation, surface traction and water dispersal standards, markings, signs and lighting. The overriding philosophy behind runway standards is to remove as many variables as possible from the routine but always potentially dangerous act of landing a large transport category aeroplane.
Pressurisation
Not having wings is only one of the ways in which humans were never designed to fly. Above about 10,000 feet, the human body begins to have issues with the reduced air pressure, which results in there not being enough oxygen in the air for our bodies to work as intended. The condition in which the body does not get enough oxygen is called hypoxia, and gradually moves from a curious case of altitude sickness to unconsciousness and then death, depending on the severity of the oxygen deprivation.. How then do passengers on commercial aircraft, which routinely fly at up to 40,000 ft, survive? The answer is pressurisation: treating the fuselage essentially like a can of soft drink, that is, the fuselage becomes a pressurised vessel. Air is ‘bled’ off the jet engine (or the turbocharger in pressurised piston-engine aircraft) and used to pressurise the aircraft. Most commercial aircraft have pressurised fuselages that simulate an atmospheric pressure of around 8000 ft. The first aircraft to feature a pressurised cabin was the Boeing 307, introduced in 1940. Pressurisation has the added benefit of enhanced passenger comfort at higher altitudes – ‘above the weather,’ as 1960s airline advertising used to say.
Pitot-static covers
In February 1996, Birgenair Flight 301 was preparing for take-off. The Boeing 757 had not been flown for almost a month; during which time the pitot-static tubes had not been covered. During take-off the pilot’s air speed indicator was reading fast while the first officer’s was reading normally. When the autopilot was engaged a few minutes later it tried to counter the apparent overspeed by reducing power and pitching the aircraft nose up. In fact, the aircraft was dangerously under speed and overpitched, a condition that set off the aircraft’s stick-shaker stall alert. This confused the pilot, who delayed the correct response of applying power and pushing the nose down. The aircraft crashed around five minutes after take-off, killing 189. Investigations concluded a wasp had built its nest inside the pitot tube during the aircraft’s extended stay on the ground. This is why aircraft have brightly coloured pitot-static covers put over the pitot-static tubes to protect them from debris (including insects), when they are on the ground for an extended time. This is a safety-critical issue; the loss of pitot-static information will prevent an aircraft from receiving correct data on airspeed and altitude, reducing situational awareness and safety.
Ground-based radio navigation
Radio can be used to determine an aircraft’s heading. This is facilitated by ground- based radio stations, which send out directed radio signals that an aircraft can receive and use to determine its position relative to the radio source. Since World War I, radio direction finding (RDF) has been recognised as very valuable in determining the position of enemy targets. The problem was that the station would identify its own position relative to the threat, while in aviation, the ‘threat’, or aircraft, needed to be able to ‘find’ the station. Innovation created smaller and smaller radio equipment, allowing aircraft to reverse RDF and locate ground-based stations, the early beginnings of the VOR system. Today the very high frequency omnidirectional radio (VOR) system is used to determine an aircraft’s bearing by radio. However, with the surge in popularity of GPS-based navigation VORs are beginning to be phased out due to their high maintenance costs.
Phonetic alphabet
Unambiguous communication is imperative to aviation safety but English, the international language of aviation, is highly ambiguous. For example, the letters B, C and D can sound alike when heard under stress and over distance on a crackly radio. Enter the ICAO phonetic alphabet. It was developed in the 1950s to represent the letters of English without using any vocal sounds unique to English.
Allied forces in World War I used the first spelling alphabet, which continued to evolve throughout World War II with variations between the British and American versions. After the war, the International Air Transport Association (IATA) recognised the need for a universal approach to safeguard against confusion and miscommunication. Taking on board IATA’s recommendations, the International Civil Aviation Organization (ICAO) further developed the alphabet to its current form:
Alfa, Bravo, Charlie, Delta, Echo, Foxtrot, Golf, Hotel, India, Juliett, Kilo, Lima, Mike, November, Oscar, Papa, Quebec, Romeo, Sierra, Tango, Uniform, Victor, Whiskey, X-ray, Yankee, Zulu.
Bonus for pedants: Strictly speaking it is not a phonetic alphabet, like the series of symbols linguists use to describe the sounds in a language or dialect, but a spelling alphabet.
Radar
Radio detection and ranging (radar) was developed by several nations during World War II to help track the altitude, direction and speed of aircraft and ships. Today it is widely used throughout society and has underpinned major advances in aviation safety. There are two types of radar. Primary surveillance radar (PSR) transmits a pulse of energy that is reflected back to the radar dish. This allows the direction and distance of the aircraft to be calculated and displayed. Secondary surveillance radar (SSR) transmits an interrogation signal to which the aircraft’s transponder replies with the aircraft’s assigned ID (mode A), its altitude (mode C), or more comprehensive aircraft data via mode S. The two systems are distinct. Primary radar detects aircraft, whether they wish to be detected or not, but cannot tell much about what it sees. It can be fooled by stealthy signal-absorbing design or, historically, by scattering reflective foil from an aircraft, as the allied air forces did in World War II. Secondary radar is a cooperative system: if cooperation is withdrawn by switching off the transponder, secondary radar is blind. Secondary radar enables air traffic control (ATC) to track and coordinate aircraft over busy aerodromes and airports. All high-capacity aircraft and many smaller aircraft also have radar transponders. Modern secondary radar displays include conflict alert software to reduce the risk of aircraft collisions. This is in addition to airborne traffic collision avoidance systems (TCAS) that are independent of ground radar returns. Primary radar, both on the ground and in aircraft, also plays an important role in forecasting and avoiding severe weather.
Safety cards
The first passenger briefing cards (produced in the 1930s) were mostly in the written word, while today’s cards usually only have pictograms and icons. The early cards tended to be amateurish and hard to decipher and the information was not always reliable – some even showed the emergency exits in the wrong place! During the 1960s, more complete safety measures were explained in separate safety booklets, then during the 1970s, cards became increasingly precise and efficient, and smaller airlines began to adopt them.
All commercial aircraft are now equipped with aircraft safety cards illustrating procedures such as buckling seat belts, bracing for impact in a crash, dealing with depressurisation, opening emergency exit doors, or using slides and flotation devices in the event of a ditching. Pre-flight safety demonstrations (either by the flight attendants or as a video presentation) reinforce the safety messages and remind passengers to familiarise themselves with the safety cards before take-off.
The cards are usually laminated or made of plastic and have instructions specific to particular aircraft types. The graphic representation makes the information accessible to children, speakers of different languages and passengers who cannot read.
The NZ Civil Aviation Authority produces passenger safety briefing card kits that can be customised for various aircraft types and the Air Safety Institute has a useful video on safety briefings in smaller aircraft.
Interestingly, aircraft safety cards are now popular collectibles, considered to be unique examples of everyday information graphics that reflect airlines, aircraft types, cultures, and historic periods.