On a dark night, a pilot begins an unnecessary fight with the helicopter’s autopilot, and loses
By Brendan Reinhardt
In the Disney film Fantasia, Mickey Mouse is a sorcerer’s apprentice (based on a German fable) who borrows his master’s magical cap to help him move pails of water by enchanting a broom.
Things go well enough initially; however, without fully understanding or being properly trained in the magical powers he now controls, things soon get out of hand and the broom moves too much water.
The apprentice then ends up fighting the broom and cutting it into little pieces but this only makes things worse, as more and more brooms now move more and more water until the room begins to flood. The more Mickey fights the brooms that are meant to be helping, the worse the situation becomes.
Merlin helicopter
The Merlin helicopter was built to replace the venerable Sea King in the anti-submarine warfare role for the Royal Navy. The Merlin was specifically designed to hover over water at night in dark conditions.
To achieve this role and reduce pilot workload, the manufacturer equipped the helicopter with an automatic flight control system (AFCS). This allowed stability augmentation, attitude hold, automated hover and transition modes.
The Royal Canadian Air Force operated the Merlin as the CH-149 Cormorant. In 2006, one of these helicopters, call sign Tusker 914, was conducting a routine night winch training sortie to a ship.
The crew lost control and the helicopter crashed into the ocean. The cockpit structure broke away from the fuselage and the pilots managed to escape. Unfortunately, three of the seven crew died as they could not escape from the rear cabin which was underwater.
The report into the accident found the pilot had low currency and had removed his night-vision goggles to fly the final hover to the ship unaided. But as the mission was overwater and at night, this action would have reduced the horizon cues available to set an attitude.
Flying unaided to the boat would have also reduced the water-surface cues related to the helicopter movement, as it is likely that the boat and helicopter wash would have given inconsistent cues related to overall helicopter motion.
The helicopter AFCS was used with transition, hover and height-hold modes. As the helicopter approached the ship, it drifted up and down in height. A rate of descent of 500 fpm was generated at 110 feet above the water, and then the helicopter descended through 80 feet. At this point, the go-around mode was selected (transition up), however, the helicopter attitude pitched nose down to 24 degrees before recovering to 18 degrees and torque exceeded 100 per cent.
The analysis
The flying pilot was fighting the AFCS, manually pushing the cyclic through the autopilot and utilising the trim release switches, both of which interfered with the ability of the AFCS to operate the upper modes that had been selected.
The automatic flight control system was perfectly capable of keeping the helicopter stable and fly it away once commanded. However, in this accident, the fight between the AFCS and the pilot eventually flew the helicopter into the ocean at 18 degrees nose down, 69 knots and 800 fpm.
There is much in this accident that is worth studying for anyone who operates over water or is challenged by introduction-to-service issues for newer aircraft. If this is you, then it is certainly worth benchmarking your operations against this accident.
However, here we will focus only on the use of the AFCS and the useable cue environment (UCE). This concept is described in Aeronautical Design Standard-33 (ADS-33) which outlines in part how to design acceptable flying qualities for helicopters.
To improve flying qualities, modern helicopter design introduces high levels of automation with AFCS being used to reduce pilot workload. The other half of the equation to achieve good flying qualities is how well the pilot perceives motion with reference to the available cues, or the UCE.
The fight between the AFCS and the pilot eventually flew the helicopter into the ocean at 18 degrees nose down, 69 knots and 800 fpm.
This can be determined by assessing the available attitude, horizontal translation and vertical translation cues. Numeric scores allow standardised grading of the overall UCE. This process involves using Figure 1 and then Figure 2 to get the UCE.
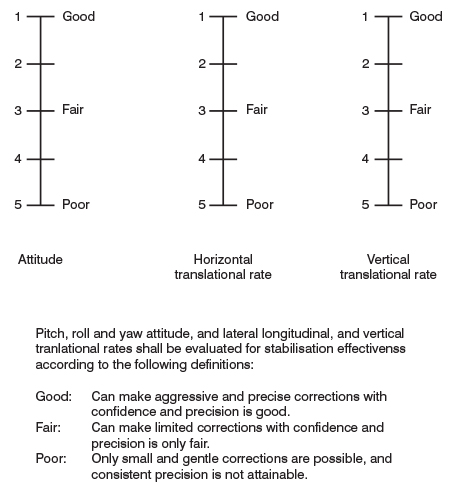
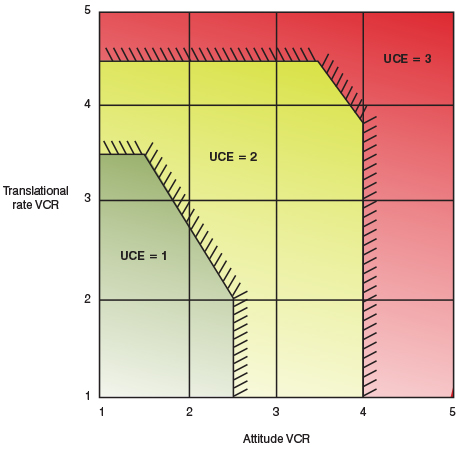
As the UCE gets worse (1 to 2 then 3), higher levels of automation are required to safely fly the aircraft. ADS-33 specifies the level of AFCS automation required to operate in a given cue environment. The better the environment, the less automation required to achieve acceptable handling qualities.
Modern automatic flight control systems will have a stability augmentation system (SAS) that provides fast-acting, short-term stability. This is helped by longer-term, slower-acting systems that maintain the aircraft attitude.
This provides the pilot with a basic level of automation where disturbances due to gusts are reduced and the aircraft maintains the attitude that is set. Normally a heading hold for hover and turn coordination for forward flight are also incorporated.
In the hover, the next level is basic position and height holding, normally provided by a doppler or radar altimeter. Then, the aircraft will automatically maintain a stable hover position at a nominated height.
The final level of automation provided is translational rate command where (sometimes through the trim hat) the pilot can command horizontal velocities while in the automated hover.
All these AFCS modes are designed to be used in different UCE, and ADS-33 outlines this.
The automatic flight control system was perfectly capable of keeping the helicopter stable and fly it away once commanded.
With good attitude (visual horizon) and position cues (accurate microsurface texture), the pilot can hover the aircraft utilising the basic SAS with an acceptable workload. The cues are rich and direct and changes can be seen easily and quickly with corrections applied in a timely manner.
UCE 1 could be considered good daylight conditions. As this degrades, the specification outlines how the aircraft is designed to be hovered with attitude hold, heading hold and height hold.
As the pilot cannot see good horizon cues or the surface texture delays position cues, then more AFCS automation must be relied on. UCE 2 might be overcast conditions, cloud onto the surrounding hills with rain and poor surface texture cues such as a smooth uniform dry lake bed. It could also be on a bright moon night, utilising NVD with poor surface texture cues.
In UCE 3 there are very poor horizon and surface texture cues, requiring full automation to allow the pilot to safely control the helicopter.
Hovering over a glassy ocean without a good visual horizon could also be considered a UCE 3 environment. Similarly, hovering over a low-contrast salt pan on a very dark misty night may also provide very little attitude and position cues and also be considered UCE 3.
Here, the pilot is unlikely to perceive changes in the aircraft attitude, vertical and horizontal position until the magnitude of change is large – the workload to fly the aircraft becomes unmanageable.
Each helicopter has subtle differences in exactly how its AFCS operates. Entry parameters and mode changes can all be slightly different. Exactly what the force trim release switches do and what modes they remove can be slightly different between helicopters.
It is critical that if you operate in UCE 3 environments, you understand these concepts and position the aircraft to allow the AFCS to smoothly capture the desire mode.
If the system has been given every chance to correctly capture a mode and is struggling due to turbulence or power limits, then the answer is not necessarily to hand fly the aircraft if in UCE 3.
If you don’t have the useable cues to accurately and quickly perceive changes in attitude and position, you are probably going to have a worse time than the autopilot. If the autopilot can’t fly the aircraft in UCE3, then it is probably time to reposition for less turbulence or a different approach angle without crosswind, reduce weight or head home.
If the autopilot fails in UCE 3 conditions, it is probably wisest to go home. In such an environment, the pilot cannot rely on their perception of the visual cues and, even if they are accurate, the pilot has probably perceived the change too late. In UCE 2 and 3 environments, the helicopter is designed to be flown with the help of the autopilot.
The lack of understanding how the AFCS works and how to utilise it, combined with poor attitude and position cues, meant that in the 2006 accident, an aircraft that can comfortably hover at low level all night long without the pilot virtually seeing anything, flew itself into the water.
In this case the sorcerer’s or Merlin’s apprentice did not fully understand, nor had they mastered the knowledge, to safely operate the magical powers of a modern helicopter’s automatic flight control system.
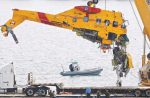
*In Australia on 13 May 2018, an AW139 helicopter came within 31 feet of terrain near Darwin, at night in low UCE.